The year was 1953. Dwight Eisenhower was president, Burt Lancaster and Deborah Kerr were rolling around on the beach in From Here to Eternity, and engineers in Flint, Michigan, put the finishing touches on the first-ever Chevrolet Corvette.
About 225 miles north, two parallel 20-inch pipelines were getting their first taste of crude oil on the bed of the Straits of Mackinac. Designed by the Bechtel Corporation—a company made famous by building the Hoover Dam 20 years prior—the Straits Pipelines stretch out for 4.5 miles, tethered to the bottom of the channel by screw anchors at depths ranging from 65 to 270 feet. They are part of a 645-mile pipeline system known simply as Line 5. Line 5 runs from Superior, Wisconsin, to Sarnia, Ontario, Canada, and is now owned and operated by multinational energy corporation Enbridge.
At the time of the Straits Pipelines’ commissioning, the State of Michigan included language in the construction easement that required the pipelines’ owner to maintain an adequate financial assurance mechanism— such as insurance, a trust fund, or a surety bond—to cover liability for all damages or losses in the event of a worst-case-scenario. The easement did not predict what such costs would be, other than to say they would total “at least” $1 million.
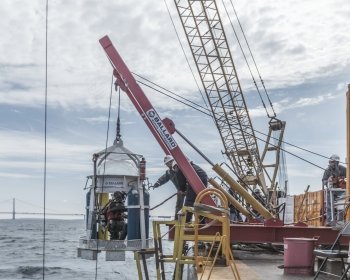
Fast forward to July 26, 2010, when Michigan experienced one of the largest inland oil spills in US history. A pipeline known as Line 6B burst and spilled heavy crude into a tributary of the Kalamazoo River. In the spill’s wake, national attention focused on oil transportation safety, including that of Line 5.
In 2015, the Michigan Petroleum Pipeline Task Force, a state multi-agency group established after the 2010 spill, issued a report that made 13 recommendations, including the establishment of a Pipeline Safety Advisory Board (PSAB) and the commissioning of two studies: a risk analysis and an alternatives analysis for the Straits Pipelines portion of Line 5.
The final alternatives analysis was released in November 2017. But the independent risk analysis, which was scheduled to be completed at the same time, took a detour and arrived on Michigan Tech’s doorstep.
Complex Flows
In 2016, after an extensive request-for-proposal process, the State of Michigan hired Det Norske Veritas (DNV), a Norwegian company, to conduct an independent risk analysis of the Straits Pipelines. A few days before the release of DNV’s draft report, however, the State terminated its contract, citing a conflict of interest. The public called for the immediate hire of a replacement contractor, and the State agreed.
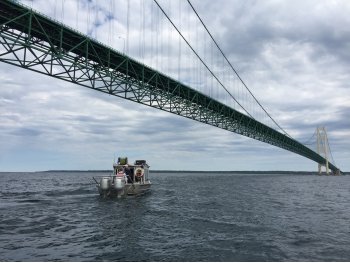
At the time, Guy Meadows, director of Michigan Tech’s Great Lakes Research Center (GLRC), served as the PSAB’s state university representative. When looking to replace DNV, Meadows says, the State wanted to find an unbiased group that understood the full picture.
Michigan’s universities were the answer.
“There was a suggestion that Michigan Tech should lead the process because of our extensive knowledge of the Straits of Mackinac region and its complex flows,” Meadows says. When the University’s name came up for consideration, Meadows recused himself from voting; on September 17, 2017, the PSAB voted unanimously to place Michigan Tech at the helm of a new risk analysis.
All Hands on Deck
For the next three months, Meadows, Elizabeth Hoy ‘96, assistant director of business and program development for the GLRC, and Amanda Grimm, research scientist and geospatial researcher at the Michigan Tech Research Institute (MTRI) in Ann Arbor, worked around the clock to assemble the risk analysis team and put together a proposal for the State’s review.
“While Michigan Tech was asked to lead the project,” Meadows says, “what the State wanted was for Michigan universities to work together to provide a science-based assessment of the true costs of a major spill.”
“The scope of work required a broad distribution of people,” Hoy says. “Michigan Tech’s Vice President for Research Dave Reed, reached out to his counterparts at the other state universities, and we got some great responses. From there, we asked whether there were other capabilities and areas of expertise that we needed. We did some research and approached the experts. Very few people on the project knew each other beforehand.”
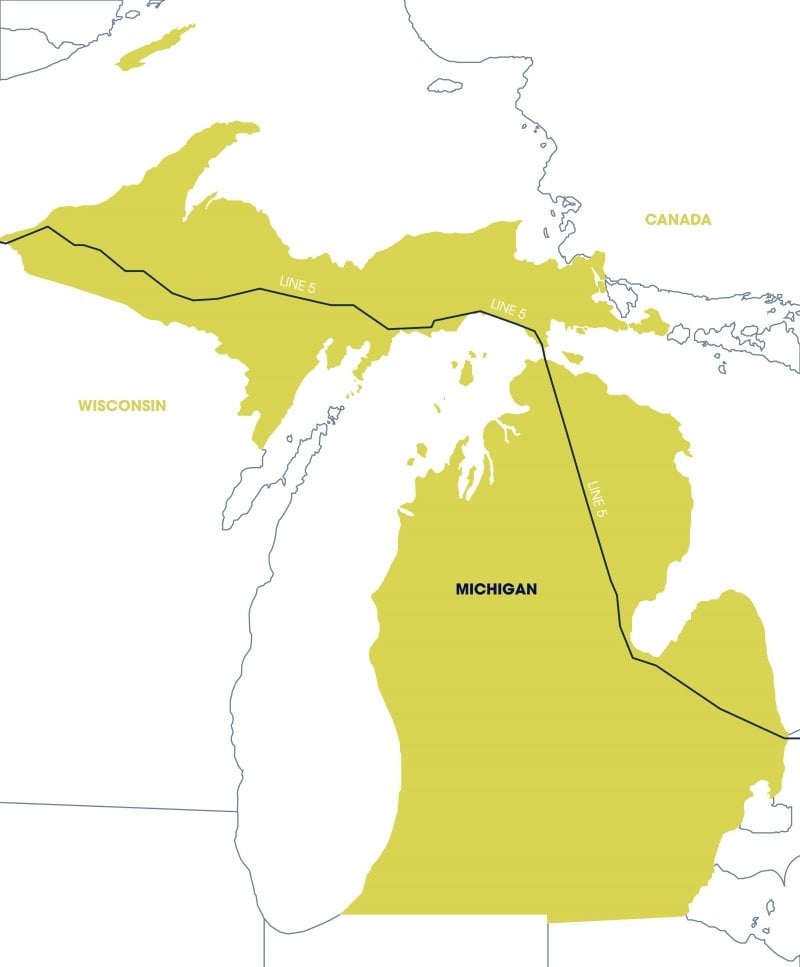
On December 11, 2017, Meadows gave the PSAB an overview of his proposal, built around a team of 41 researchers—21 from Michigan Tech and 20 from external organizations. Of the nine universities that would contribute to the analysis, seven were within the state of Michigan: Michigan Tech, the University of Michigan, Michigan State University, Wayne State University, Western Michigan University, Grand Valley State University, and Oakland University. The two out-of-state universities were North Dakota State University and Loyola University Chicago.
Three researchers on the project were from consulting organizations, and two were independent contractors. Two other contributors, both from the National Oceanic and Atmospheric Administration (NOAA) Great Lakes Environmental Research Laboratory, would donate their time and expertise in numerical modeling.
The State accepted the proposal, and the team officially began its work on January 15, 2018.
Defining the Worst
In tackling the risk analysis, each section of the report had a section leader, a chief scientist, and at least two section authors. Grimm served as section lead for Task A, identifying the worst-case-scenario.
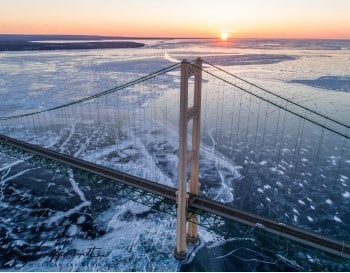
“By definition, the worst-case spill size is much larger than what would be expected under average or typical conditions,” Grimm says. Researchers based their approach on an accumulation of worst-case assumptions that were consistent with the federal definition of “the largest foreseeable discharge of oil,” found in Title 40, section 194.5 of the Federal Code of Regulations, to determine the maximum possible volume that could be released.
According to the draft report, the impacts of a spill depend on when it occurs and how meteorological conditions disperse the oil.
“A spill in the spring, just prior to the summer tourism season in the Straits, would have the largest economic impact overall,” Grimm says. “We needed to follow one scenario all the way through various monetary costs that would be incurred to come up with the total amount of financial liability.”
Because no oil spill as large as the scenarios analyzed in the report has ever occurred in the waters of the Great Lakes, researchers reviewed a selection of other events as analogs to evaluate the potential impacts of a Straits spill. The report also considers various modes of failure for the Straits Pipelines and separates these failures into five tiers. Under this model, discharge amounts range from 4,400 barrels of oil (bbl) to 58,000 bbl. With each bbl containing 42 US gallons, the discharge amount could reach nearly 2.5 million gallons of oil.
Assessing the Worst
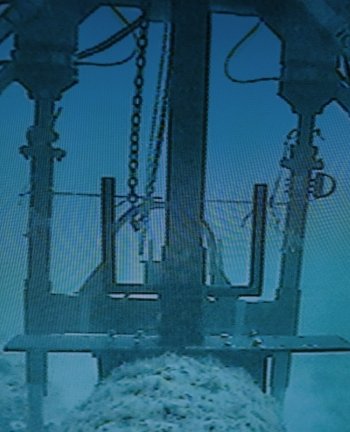
In addition to her role as Task A’s lead, Grimm wore two other hats, serving as the project’s day-to-day coordinator and its data manager. Due to the project’s short timeline, researchers would not have the time to accumulate new data, so Grimm tracked down more than 60 existing data sets, on everything from gill net surveys to Mackinac Bridge traffic volume. She and Ryan Williams, a geospatial research scientist at the GLRC and assistant director at the Michigan Tech geospatial research facility, worked with colleagues to create web maps that helped team members work together remotely. Some of the most critical existing data resided with researchers at NOAA—a hydrodynamics data set from 2016 that included water movement and meteorological conditions for the entire year. The information set was so extensive that the NOAA contributors had to purchase external hard drives, upload the data, and overnight the hard drives to Houghton.
“We received about two terabytes of data,” Grimm says. “There just isn’t cloud capacity for that amount of material.” A terabyte is approximately one trillion bytes of data.
Michigan Tech’s Pengfei Xue, chief scientist for Task B (analyzing the likely environmental fate and transport of oil or other products released from the Straits Pipelines in a worst-case scenario), used NOAA’s hydrodynamics inputs and an oil dispersal model to run the more than 4,300 simulations of how a spill might affect the Straits.
One of the more than 4,300 oil dispersal simulations run by Xue's team. The animation shows floating particles in red moving through the water and beached particles along the shore in green. Ending the simulation, at 1,435 hours after release, 99.8% of particles were beached.
"Guy Meadows and his team of researchers brought great professionalism and rigor to the independent risk analysis. Dr. Meadows led a team of top researchers from nine universities on this important task. Thanks to his leadership and effort, their work became a critical part of the State’s evaluation of the Line 5 pipeline in the Straits of Mackinac, helping us to chart the best path forward for the Great Lakes and for Michigan."
Xue, who is the director of the GLRC’s Numerical Geophysical Fluid Dynamics Laboratory and an assistant professor of civil and environmental engineering, was a core member of the development team for the hydrodynamic model (Finite Volume Community Ocean Model or FVCOM) and the associated particle-tracking model used in this project, so he felt right at home running simulations of oil dispersal in the Great Lakes.
The Task B team ran their 4,380 simulations on Superior, the University’s shared high-performing computing infrastructure (or supercomputer cluster). Superior is one of the most powerful high-performance computer clusters in the region.
- PI = Principal Investigator
- CS = Chief Scientist
- SL = Section Lead
- SA = Section Author
- MTU—Michigan Technological University
- GVSU—Grand Valley State University
- OU—Oakland University
- UM—University of Michigan
- WMU—Western Michigan University
- WSU—Wayne State University
- LUC—Loyola University Chicago
- NDSU—North Dakota State University
- NOAA GLERL—NOAA Great Lakes Environmental Research Laboratory
- PASS—Powell and Associates
- DoE—Department of Energy
- AFPM—American Fuel and Petrochemical Manufacturers
- [see accessible version]
“Movement of oil in the water depends on the water itself, ice, wind conditions, and other weathering processes, such as evaporation,” says Xue, explaining that in a Lagrangian particle-tracking model a cloud of numerical tracers represents an oil spill, and that cloud shifts and migrates depending on different conditions. “We ran each simulation with oil being allowed to disperse for a maximum of 60 days, at six-hour intervals throughout the whole year. From these simulations, we could examine scenarios under a set of metrics including length of oiled shoreline, area of oiled open water, and volume of beached oil. So each task team could choose worst-case scenarios best suited for their area of study.”
When all was said and done, the Task B team produced approximately eight terabytes of data, repackaged visually for the rest of the team. The simulation results updated previous oil dispersal modeling capabilities for the Great Lakes to include potential effects of wind, ice-cover, and evaporation on oil dispersal.
Meadows and Xue explain that ice cover alters the trajectory of oil movements. In the winter, ice slows oil in reaching the shoreline and allows oil to stay in the water for a longer period of time. In the case of the Straits of Mackinac, this means a higher possibility that oil from a ruptured pipeline would reach both Lake Michigan and Lake Huron. While the effect of ice cover could allow more oil to be recovered before reaching the shoreline, it would also likely mean a longer response time and lengthier period of cleanup. The harsher the winter conditions, the harder it would be to clean up a spill. And if responders are unable to take immediate action, the oil would spread to an even greater extent.
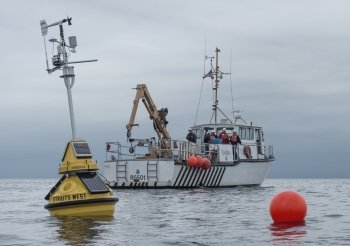
Task B team member Dave Schwab, a world-renowned research scientist and hydrodynamic modeling expert from NOAA, now at the University of Michigan’s Graham Sustainability Institute and MTRI, notes that the work done in relation to the risk analysis advanced the state of the art in numerical modeling for the Great Lakes. Schwab was one of the original designers of NOAA’s Great Lakes Coastal Forecasting System in the 1990s and says he has witnessed continuing remarkable improvements in computer modeling of the Great Lakes since then.
“Great Lakes monitoring and prediction systems, critical to the large percentage of US and Canadian populations in the region, often precede similar ocean monitoring systems by a decade,” Schwab says. “As part of the Line 5 risk analysis, we were able to include the effect of ice cover on circulation in the Straits of Mackinac for the first time. This modeling improvement will also be incorporated into NOAA’s operation Great Lakes forecasting system.”
Xue notes that the models produced in this project served as a layer that impacted all the other tasks. Indeed, teams working on other sections of the report used the simulation results to run their own analyses, including public health and safety impacts, ecological impacts, and natural resource damages. Those impacts and damages provided the basis for the $1.8 billion figure the team’s economists estimated total worst-case-scenario costs to be.
Beyond the Straits
The report has had an immediate impact on the Great Lakes region. It highlighted impacts on Ontario and Wisconsin in ways previous reports did not, and it factored heavily into the State of Michigan’s October 3, 2018, agreement with Enbridge to permanently shut down the Straits Pipelines and construct a multi-use utility tunnel beneath the Straits.
Members of the risk analysis team believe the report’s impact will also extend well beyond nearby shores. “Our work pointed out knowledge gaps,” Grimm says. “In some areas, we had to make assumptions about, for example, crude oil in a freshwater system because there was no historical open freshwater spill similar enough to use as a model. That kind of information gap has become a research need— how does oil behave in large freshwater systems? That should become more widely known.”
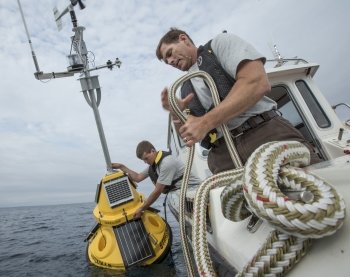
Grimm also notes that the risk analysis can inform other governments as to what consequences to consider when proactively planning response efforts to smaller hydrocarbon spills.
Xue notes that visualizing model results helps create immediate understanding for a non-technical audience.
“People are becoming more familiar with what modeling is, how it works, and how it can help the public and state governments address the ‘what if’ question,” he says, explaining that a visual “gives people a take-home message about how big the impact could be. Similar models can be used to help people understand and make decisions about things like climate change, and to study the transport and fate of other contaminants, like algal blooms.”
Cooperative Collaboration
Hoy and Meadows are amazed by what the researchers were able to accomplish in a six-month timeframe.
“This project came together quickly with little notice,” Hoy says. “People took on the responsibility of their tasks and roles in addition to the things that they were already doing in their day job—teaching, research projects, etc. Everyone who participated stepped above and beyond to accommodate this important project.” Meadows agrees. “The universities and NOAA, our federal partner, came together to provide a highly technical analysis of a very complex, multifaceted problem for the state of Michigan. The people who participated agreed with the purpose of the report. They were hardworking, and they did fine work.”
Meadows also notes the key role that the State of Michigan and the public played in the process. “Their feedback and comments were an integral, important part of the process.”
Michigan Technological University is a public research university founded in 1885 in Houghton, Michigan, and is home to more than 7,000 students from 55 countries around the world. Consistently ranked among the best universities in the country for return on investment, Michigan’s flagship technological university offers more than 120 undergraduate and graduate degree programs in science and technology, engineering, computing, forestry, business and economics, health professions, humanities, mathematics, social sciences, and the arts. The rural campus is situated just miles from Lake Superior in Michigan's Upper Peninsula, offering year-round opportunities for outdoor adventure.